Functional and Radiological Outcomes of All-Posterior Surgical Correction of Dystrophic Curves in Patients with Neurofibromatosis Type 1
-
Ajoy Prasad Shetty
, Jalaj Meena
, Chandhan Murugan
, Rounak Milton
, Rishi Mugesh Kanna
, Shanmuganathan Rajasekaran
- Received August 27, 2023 Revised December 12, 2023 Accepted December 14, 2023
- ABSTRACT
-
- Study Design
- A retrospective cohort study.
- Purpose
- To determine outcomes following all-posterior surgery using computed tomography navigation, hybrid stabilization, and multiple anchor point techniques in patients with neurofibromatosis type 1 (NF-1) and dystrophic scoliosis.
- Overview of Literature
- Previous studies favored antero-posterior fusion as the most reliable method; however, approaching the spine anteriorly was fraught with significant complications. With the advent of computer assisted navigation and multiple anchor point method, posterior only approach is reporting successful outcomes.
- Methods
- This study included patients who underwent all-posterior surgical deformity correction for dystrophic NF-1 curves. Coronal and sagittal Cobbs angles, apical rotation, and the presence of dystrophic features were evaluated before surgery. Postoperatively, sagittal, coronal, and axial correction, implant position, and implant densities were evaluated. The decline in curve correction and implant-related complications were evaluated at follow-up. Clinical outcomes were evaluated using the Scoliosis Research Society-22 revised index.
- Results
- This study involved 50 patients with a mean age of 13.6 years and a mean follow-up duration of 5.52 years. With a mean coronal flexibility of 18.7%, the mean apical vertebral rotation (AVR), preoperative coronal Cobb angle, and sagittal kyphosis were 27.4°, 64.01°, and 47.70°, respectively. The postoperative mean coronal Cobb angle was 30.17° (p<0.05), and the sagittal kyphosis angle was 25.4° (p<0.05). The average AVR correction rate was 41.3%. The correction remained significant at the final mean follow-up, with a coronal Cobb angle of 34.14° and sagittal kyphosis of 25.02° (p<0.05). The average implant density was 1.41, with 46% of patients having a high implant density (HID). The HID had a markedly higher mean curve correction (29.30° vs. 38.05°, p<0.05) and a lower mean loss of correction (5.7° vs. 3.8°, p<0.05).
- Conclusions
- Utilizing computer-assisted navigation, hybrid instrumentation, and multiple anchor point technique and attaining high implant densities, this study demonstrates successful outcomes following posterior-only surgical correction of dystrophic scoliosis in patients with NF-1.
- Introduction
- Introduction
Spinal deformity is the most prevalent musculoskeletal manifestation of neurofibromatosis type 1 (NF-1), affecting 10%–60% of patients [1,2]. Short, acute, angular, and frequently progressive dystrophic curves necessitate early surgical intervention, including the growing rod technique and definitive fusion [3,4]. The literature suggests that anteroposterior fusion is the most reliable surgical option [5–7]. Kim and Weinstein [8] suggested anteroposterior instrumented fusion for deformities >40° or kyphotic angles >50° to improve correction efficacy and decrease the risk of pseudoarthrosis. However, the anterior approach presents several difficulties, such as the approach to a hyperkyphotic and severely rotated spine and the potential presence of a complex tumor or excessive plexiform venous channels around the vertebral bodies [9]. Furthermore, subluxated or severely rotated vertebrae impede the placement of anterior strut grafts in the concavity of the kyphosis. The all-posterior approach avoids these complications; however, early reports indicated a high failure rate [10]. Even after the introduction of segmental stabilization with pedicle screws, inserting these screws into severe deformities with extremely narrow pedicles was extremely challenging. This resulted in pedicle screws being easily misplaced and allowing fewer screws to be inserted into the pedicles of crucial vertebrae. Parisini et al. [11] reported failure rates for all-posterior fusion of 53%, compared with 23% for combined fusion. However, recent investigations using the multiple anchor point method have achieved significant success in posterior-only surgeries [12]. Failure rates were reduced to <4% as a result of the improved distribution of corrective forces. The use of computer-assisted navigation (CAN) has significantly enhanced the accuracy of pedicle screws and allowed the use of larger diameter screws, instrumentation of deformed pedicles with short pedicle screws, and costovertebral screws, thereby increasing the pullout strength and decreasing the incidence of implant failures [13,14]. The outcomes were further enhanced by hybrid stabilization using multiple anchor point techniques. Therefore, this study aimed to determine the functional and radiological outcomes of patients with NF-1 and dystrophic scoliosis who underwent all-posterior instrumented deformity correction and fusion using the multiple anchor point technique. This study also analyzed the influence of implant density on patient outcomes.
- Materials and Methods
- Materials and Methods
- 1. Surgical procedure
- 1. Surgical procedure
All patients underwent instrumented all-posterior deformity correction and fusion. With instrumentation spanning the entire length of the curve, including both end vertebrae, a long segment fusion was performed. The maximum posterior anchor point technique (MAT) was implemented, indicating that as many fixation points as feasible should be achieved in the key vertebrae to distribute the corrective torsional forces and prevent implant failures. According to Pushpa et al. [15], type 1 pedicles were instrumented with standard pedicle screws, whereas type 2 pedicles were instrumented with short pedicle screws. Either the in-out-in technique (Fig. 2) or sublaminar wires were required for type 3 pedicles. Instrumentation was performed under the guidance of an intraoperative CT (iCT)-based navigation system and a mobile AIRO CT scanner (Brainlab AG, Feldkirchen, Germany) (Fig. 3). On the concave side, soft tissue contractures were relieved, and facetectomy was performed along the entire instrumentation. The intertransverse ligaments and costotransverse articulation ligaments were also removed from the rigid segments. Parts of the ribs and transverse processes of the apical vertebrae were removed, if necessary. The deformity was corrected by contouring titanium rods and using various corrective procedures. A posterior fusion bed was prepared by decortication, and a combination of autograft and allograft obtained locally was applied. A minimum of 6 months of postoperative splints were prescribed, followed by gradual weaning. Patients with a curvature >80° underwent halo traction for 3–6 weeks until they attained the desired respiratory functional status and maximum spinal flexibility before surgery.- 2. Statistical analysis
- 2. Statistical analysis
SPSS ver. 17.0 (SPSS Inc., Chicago, IL, USA) was used to analyze the data. Parameters at the preoperative, postoperative, and ultimate follow-up stages were compared using paired t-tests. A p-value <0.05 indicated a statistically significant difference.
This retrospective observational study was conducted at Ganga Hospital, Coimbatore, India and was approved by the institutional review board (IRB reference no., 2022/10/14), permitting a waiver of informed consent because this was a retrospective observational analysis of only radiological images. The study included 62 consecutive patients with NF-1 and dystrophic curves in the thoracic and lumbar spine who underwent instrumented deformity correction and fusion between 2010 and 2019. Ten patients were excluded because they underwent a combined procedure, and two patients were lost to follow-up. Patients with spinal deformity of other causes, cervicothoracic deformities, patients with less than a minimum 3-year follow-up, those with incomplete imaging series, and those with neurological deficits (to avoid errors in objective assessment) were excluded from the study.Demographic information, including sex, age at surgery, preoperative treatment, comprehensive medical history, and physical examination results, was obtained from hospital records and the Hospital Information System. All patients underwent preoperative imaging according to our protocol, which included anteroposterior and lateral whole-spine weight-bearing radiographs, supine right- and left-side bending radiographs, traction films, computed tomography (CT), and magnetic resonance imaging (MRI). Preoperative radiographs were analyzed to ascertain the curve apices, magnitude, rotation, curve flexibility, curve type based on the presence of dystrophic features, and spinal segments involved. MRI and CT scans were analyzed to detect all dystrophic characteristics, associated dural ectasia, intraspinal tumors, and cord anomalies. The pedicles were classified according to the method described by Pushpa et al. [15] (Fig. 1). Postoperatively, patients underwent a complete spine imaging series at 3 weeks, 3 months, 6 months, 1 year, annually until skeletal maturity, and once every 2 years after that. No additional imaging was performed to assess fusion status to reduce radiation hazards to a growing spine unless the patient presented with an acute onset of back pain, radiculopathy, or implant failure on plain radiography. The postoperative radiographs were examined to evaluate the curvature correction in the sagittal (T5–T12) [12], coronal, and axial planes, alignment in the sagittal and coronal planes, implant density, and implant position. Follow-up radiographs were evaluated for decompensation, proximal junctional kyphosis, adjacent segment disease, and loss of correction in the coronal and sagittal planes. On the basis of the implant densities’ deviation from the mean, the patients were divided into high- and low-density cohorts [16]. A comparative analysis of the degree of deformity correction and the occurrence of complications was conducted between the two groups. The hospital’s picture archiving and communication system was used to retrieve all radiological images. Patients were examined for complications related to implant prominence and skin rupture. Clinical outcomes were assessed using the Scoliosis Research Society-22 revised (SRS-22r) scoring index. All radiological assessments were separately performed by an orthopedic resident and a spine fellow, which were further ratified by a senior spine surgeon.
- Results
- Results
The study included 23 male (46%) and 27 female (54%) patients aged 13.6 years (range, 6–19 years) on average, who underwent all-posterior instrumented deformity correction for NF-1 dystrophic curves. Moreover, 38 patients (76%) had a primary thoracic curve, five had a thoracolumbar curve, and seven had a lumbar curve. The thoracic curve apex was most frequently observed at T8 (n=10, 48%), followed by T7 and T9 (n=8, 16%), with a mean apical vertebral rotation (AVR) of 27.4°. The average number of vertebrae exhibiting dystrophic characteristics was 5.79, with vertebral scalloping being the most prevalent (68.75%) dystrophic feature. In addition, 30% of patients (n=16) had dural ectasia (Fig. 4). The mean preoperative coronal Cobb angle was 64.01° (range, 33.3°–110.9°), with a mean flexibility of 18.7%. The mean sagittal kyphosis angle was 47.70° (range, 11.2°–103°). Except for one patient who had a tumor on the convex side, eight patients had paraspinal tumors on the concave side. Patients with a concave tumor had a larger mean curve magnitude (73.08°, p=0.03). In eight patients who presented with a mean Cobb angle of 81.82°, preoperative halo traction was administered for a mean of 5 weeks, and the Cobb angle improved to 72.09°. Instrumentation spanned an average of 13 segmental levels (T2–L5). Three patients underwent hybrid instrumentation in which interlaminar wires were used to obtain anchor points in the periapical region of type 3 pedicles. None of the patients underwent intraspinal tumor resection or rib head excision because all patients had an intact neurological status. The postoperative mean coronal Cobb angle was 30.17° (range, 14.7°–55°; p=0.005), and the sagittal kyphosis angle was 25.4° (range, 10.7°–48°; p=0.005). The average AVR correction rate was 41.3%. Five patients exhibited concomitant thoracic hyperkyphosis (>50°) with a mean angular value of 59.66° (range, 50°–78.3°) corrected to 46.30°. The correction remained significant at the final mean follow-up of 5.52 years, with a mean coronal Cobb angle of 34.14° and sagittal kyphosis of 25.72° (p=0.05).The average implant density was 1.41, with 46% and 54% of patients having high (>1.41) and low (<1.41) implant densities, respectively. The high implant density (HID) group had a markedly higher mean curve correction (29.30° versus 38.05°, p=0.05) and a lower mean loss of correction (5.7° versus 3.8°, p=0.05). In addition, 34% (n=9) of the low implant density group experienced significant loss, whereas only 1% (n=2) of the HID group did. At a mean follow-up of 2.7 years, two patients with a mean coronal Cobb angle of 50.5° underwent reoperation due to rod fracture. These two patients had implant densities of 1 and 0.92 (mean=0.96, p=0.05), indicating a negative correlation between implant density and implant failure. No correlation was found between the preoperative Cobb angle and the failure rate of implants. Postoperatively, the mean SRS-22r score was 96. Two patients developed superficial wound dehiscence and were treated with debridement and resuturing. No intraoperative instrumentation-related complications were observed. None of the patients, during follow-up, experienced medical complications, implant prominence and skin rupture, progression or decompensation of the curve, or neurological deficit.
- Discussion
- Discussion
The presence of associated tumors that alter the vertebral anatomy and dural ectasia make surgical management of these patients extremely challenging, with a higher incidence of implant failure, wound dehiscence, loss of correction, and proximal junction kyphosis [17,18]. Rather than focusing on the degree of correction, the primary objective of surgery is to stabilize the spine, prevent the progression of deformity, prevent nerve injury, and avoid altering respiratory function. The surgical management of these patients can include growth-friendly procedures and early fusion techniques [4]. Early spinal fusion does not result in an obvious loss of trunk height because the developing curves are usually short-segment curves with limited growth potential [19].Surgical approaches remain controversial because of dystrophic vertebral deformity, very thin pedicles, osteoporosis, and rapid progression. Kim and Weinstein [8] believed that patients with dystrophic scoliotic deformities of 20°–40° and kyphotic angles of 50° could be reliably treated with posterior spinal instrumentation and fusion. In the presence of a deformity >40° or a kyphotic angle >50°, anteroposterior instrumented fusion is recommended to improve correction efficacy and reduce the risk of pseudarthrosis [20]. However, other authors reported greater challenges associated with the combined approach in young patients with severe hyperkyphosis and vertebral rotation [17,21]. The possible presence of a complex tumor or excessive plexiform venous channels around the vertebral bodies rendered the anterior approach impossible, necessitating a second attempt from the contralateral side or even abandonment of the procedure [12]. In addition, the dystrophy may have caused the apical vertebrae to subluxate or become so severely rotated that they are no longer in alignment with the remainder of the spine [22]. This misalignment prevents the anterior strut grafts from being inserted in the concavity of the kyphosis, rendering them mechanically ineffective for preventing the progression of the condition.In the early years, posterior-only fusion had a high failure rate because of the use of first- and second-generation internal fixation systems [12]. Even after the introduction of segmental stabilization with pedicle screws, placement of posterior pedicle screws in severe deformities with extremely thin pedicles was extremely difficult, resulting in screws that were easily misplaced and fewer pedicle screws placed in the key vertebrae and adjacent to these key vertebrae. According to Parisini et al. [11], the failure rates of posterior instrumented fusion alone and combined anterior and posterior fusion were 53% and 22%, respectively. With dystrophic spinal deformities accompanied by kyphosis, posterior instrumented fusion alone had a failure rate of 63%. However, the use of CAN has substantially improved the accuracy of pedicle screws and permitted the use of larger diameter screws, short screws, and costovertebral screws in deformed pedicles, thereby increasing the pullout strength and density of these implants, both of which decrease the incidence of implant failures [13].In this study, all 50 patients with dystrophic NF-1 curves possessed extremely thin pedicles. The average coronal and sagittal Cobb angles before surgery were 64.01° and 47.70°, respectively. The average implant density in this study was 1.41, which is marginally higher than that in previous studies. This was accomplished by classifying the pedicles according to Pushpa et al. [15] and utilizing the appropriate instrumentation for each pedicle type in preoperative planning. However, the coronal and sagittal Cobb angles of each patient were significantly corrected to 30° and 25°, respectively. At the final evaluation after 5.5 years, the mean loss of correction in both planes was <2°, which was not statistically significant.The patients were grouped into high and low implant densities based on the study by Li et al. [16], who had a slightly lower implant density than our study (1.41 versus 1.35). The HID group had a substantially higher mean curve correction (38.05° versus 29.30°, p=0.05) and a lower mean loss of correction (3.8° versus 5.7°, p=0.05). A statistically significant correction of the coronal deformity was found in the HID group compared with that in the low implant density group (0.30° versus 38.05°, p=0.05), with a lower loss of correction (5.70° versus 3.80°, p=0.05) at the final follow-up. Hsu et al. [22] demonstrated a 15% loss of correction in the apical region of patients with dystrophic curves treated with minimal implant density.Two patients with a mean coronal Cobb angle of 50.5° had implant failures due to rod fracture, and two patients developed superficial wound dehiscence that healed with resuturing. The other patients did not develop any additional complications. In a similar investigation. Deng et al. [12] reported positive outcomes following a posterior-only approach to the correction of dystrophic scoliosis in 31 patients with NF-1 using the multiple anchor point method. All-posterior surgical correction was performed in all patients using a third-generation internal fixation system. All patients demonstrated excellent correction of all indices, and the mean postoperative correction rate was 58.7% with a corrective loss rate of 2.3% at a final mean follow-up of 53 months. In a separate study, Park et al. [23] found that the average correction rates for the coronal and sagittal angles were 44.7% and 23.3%, respectively. Song et al. [24] reported a series of 16 patients who underwent a single-stage posterior-only operation using a pedicle screw system. The average scoliosis and kyphosis improved from 83.2° to 27.6° and 58.5° to 26.6°, respectively, with all patients attaining successful correction and fusion maintenance.The authors reporting the national trends in spinal fusion surgery for neurofibromatosis in the United States analyzed 548 patients with NF-1 who had undergone spinal fusion surgery between 2003 and 2014 [25]. The rate of posterior spinal fusion surgeries increased by 2.9 times, whereas anterior–posterior spinal fusion surgeries decreased by 2.2 times. Patients undergoing combined surgery were considerably younger and had significantly higher hospitalization durations and costs than those undergoing posterior fusion alone. This is consistent with the notion that combined fusion is a complex, multistage process.Dystrophic vertebral deformity, pedicle dysplasia, and osteoporosis make instrumentation and correction of the deformed curves difficult. In addition, dystrophic scoliosis necessitates extremely cautious exposure during surgery because of the extremely thin lamina and extremely cautious decortication for fusion because of thin and osteoporotic spinal elements [12]. Using AIRO-iCT-based navigation and hybrid instrumentation, all our study patients who underwent all-posterior instrumented deformity correction and fusion procedures had a successful outcome. Halo Gravity Traction for 3–6 weeks before undergoing the definitive surgical procedure in patients with a significant curve may improve the rate of deformity correction [26].The study may be limited by its retrospective nature and selection bias of including only patients who underwent all-posterior approaches, as this may exclude patients with more complex and severe curves, including cervicothoracic deformities and severe sagittal plane deformities.
- Conclusions
- Conclusions
In patients with NF-1 and dystrophic curves, the goal of surgery is to halt the progression of the deformity rather than to achieve a significant cosmetic correction. The use of CAN, hybrid instrumentation, and MAT has significantly improved failure rates in all-posterior surgeries for dystrophic curves while avoiding the frequent and significant complications associated with combined anterior and posterior procedures. Appropriate preoperative planning by identifying the pedicle morphometry and including adequate armamentarium and the utilization of the aforementioned techniques have enabled us to achieve satisfactory surgical correction and clinical outcomes in all-posterior surgeries in these patients.
- Notes
- Notes
-
Conflict of Interest No potential conflict of interest relevant to this article was reported.
Author Contributions Ajoy Prasad Shetty: conception and design, acquisition, analysis, and interpretation of data, critical revision of the manuscript for important intellectual content, supervision. Jalaj Meena: acquisition, analysis, and interpretation of data. Chandhan Murugan: analysis and interpretation of data, drafting of the manuscript. Rounak Milton: analysis and interpretation of data, drafting of the manuscript. Rishi Mugesh Kanna: conception and design, acquisition, analysis and interpretation of data, critical revision of the manuscript for important intellectual content. Shanmuganathan Rajasekaran: conception and design, acquisition, analysis and interpretation of data, critical revision of the manuscript for important intellectual content, supervision.
Fig. 1

Fig. 2
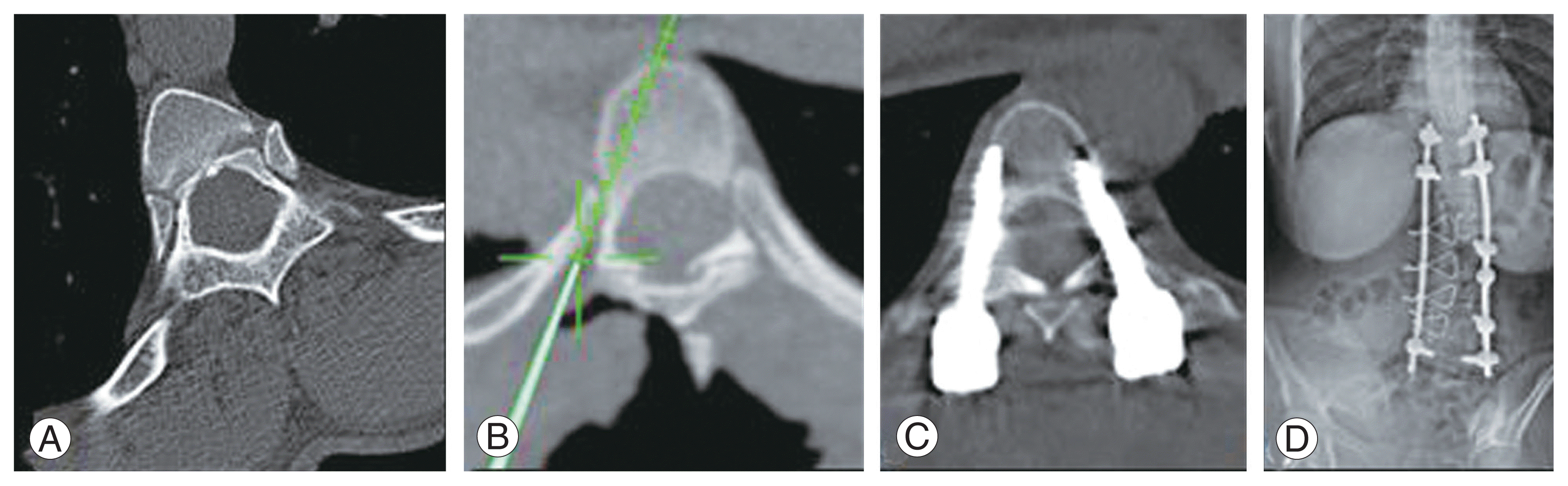
Fig. 3
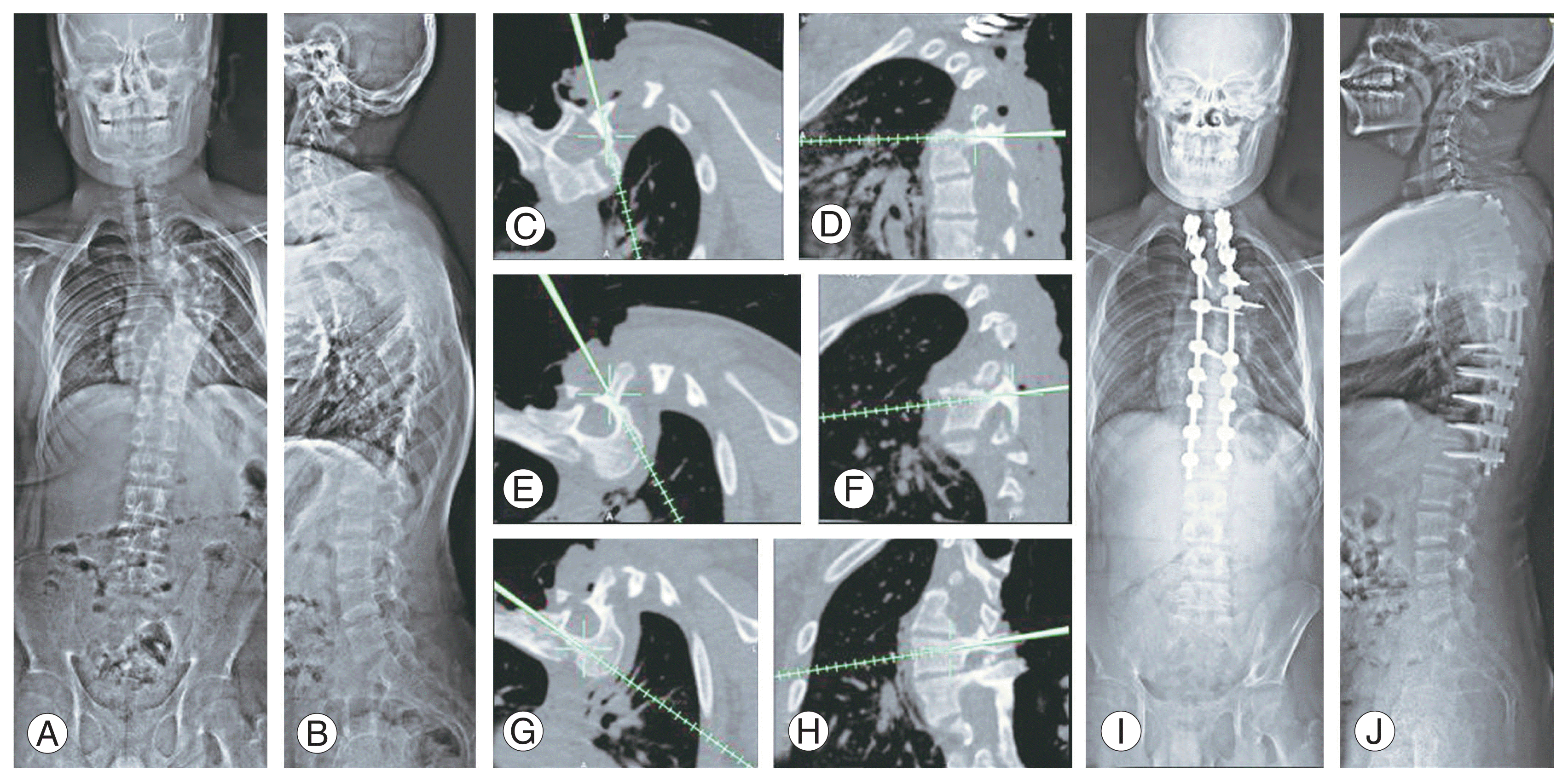
Fig. 4

- REFERENCES
- REFERENCES
References
1. Chaglassian JH, Riseborough EJ, Hall JE. Neurofibromatous scoliosis: natural history and results of treatment in thirty-seven cases. J Bone Joint Surg Am 1976;58:695–702.
[Article] [PubMed]2. Crawford AH, Bagamery N. Osseous manifestations of neurofibromatosis in childhood. J Pediatr Orthop 1986;6:72–88.
[Article] [PubMed]3. Tauchi R, Kawakami N, Castro MA, et al. Long-term surgical outcomes after early definitive spinal fusion for early-onset scoliosis with neurofibromatosis type 1 at mean follow-up of 14 years. J Pediatr Orthop 2020;40:42–7.
[Article] [PubMed]4. Cai S, Cui L, Qiu G, Shen J, Zhang J. Comparison between surgical fusion and the growing-rod technique for early-onset neurofibromatosis type-1 dystrophic scoliosis. BMC Musculoskelet Disord 2020;21:455.
[Article] [PubMed] [PMC]5. Tsirikos AI, Saifuddin A, Noordeen MH. Spinal deformity in neurofibromatosis type-1: diagnosis and treatment. Eur Spine J 2005;14:427–39.
[Article] [PubMed] [PMC]6. Halmai V, Doman I, de Jonge T, Illes T. Surgical treatment of spinal deformities associated with neurofibromatosis type 1: report of 12 cases. J Neurosurg 2002;97(3 Suppl): 310–6.
[PubMed]7. Greggi T, Martikos K. Surgical treatment of early onset scoliosis in neurofibromatosis. Stud Health Technol Inform 2012;176:330–3.
[PubMed]8. Kim HW, Weinstein SL. Spine update. The management of scoliosis in neurofibromatosis. Spine (Phila Pa 1976) 1997;22:2770–6.
[PubMed]9. Crawford AH. Pitfalls of spinal deformities associated with neurofibromatosis in children. Clin Orthop Relat Res 1989;(245): 29–42.
[Article]10. Li M, Fang X, Li Y, Ni J, Gu S, Zhu X. Successful use of posterior instrumented spinal fusion alone for scoliosis in 19 patients with neurofibromatosis type-1 followed up for at least 25 months. Arch Orthop Trauma Surg 2009;129:915–21.
[Article] [PubMed]11. Parisini P, Di Silvestre M, Greggi T, Paderni S, Cervellati S, Savini R. Surgical correction of dystrophic spinal curves in neurofibromatosis: a review of 56 patients. Spine (Phila Pa 1976) 1999;24:2247–53.
[PubMed]12. Deng A, Zhang HQ, Tang MX, Liu SH, Wang YX, Gao QL. Posterior-only surgical correction of dystrophic scoliosis in 31 patients with neurofibromatosis type 1 using the multiple anchor point method. J Neurosurg Pediatr 2017;19:96–101.
[Article] [PubMed]13. Gelalis ID, Paschos NK, Pakos EE, et al. Accuracy of pedicle screw placement: a systematic review of prospective in vivo studies comparing free hand, fluoroscopy guidance and navigation techniques. Eur Spine J 2012;21:247–55.
[Article] [PubMed] [PMC]14. Rajasekaran S, Bhushan M, Aiyer S, Kanna R, Shetty AP. Accuracy of pedicle screw insertion by AIRO(R) intraoperative CT in complex spinal deformity assessed by a new classification based on technical complexity of screw insertion. Eur Spine J 2018;27:2339–47.
[Article] [PubMed]15. Pushpa BT, Rajasekaran S, Anand KS, Shetty AP, Kanna RM. Anatomical changes in vertebra in dystrophic scoliosis due to neurofibromatosis and its implications on surgical safety. Spine Deform 2022;10:159–67.
[Article] [PubMed]16. Li Y, Yuan X, Sha S, et al. Effect of higher implant density on curve correction in dystrophic thoracic scoliosis secondary to neurofibromatosis type 1. J Neurosurg Pediatr 2017;20:371–7.
[Article] [PubMed]17. Crawford AH, Schorry EK. Neurofibromatosis update. J Pediatr Orthop 2006;26:413–23.
[Article] [PubMed]18. Crawford AH, Herrera-Soto J. Scoliosis associated with neurofibromatosis. Orthop Clin North Am 2007;38:553–62.
[Article] [PubMed]19. Jain VV, Berry CA, Crawford AH, Emans JB, Sponseller PD, Growing Spine Study Group. Growing rods are an effective fusionless method of controlling early-onset scoliosis associated with neurofibromatosis type 1 (NF1): a multicenter retrospective case series. J Pediatr Orthop 2017;37:e612–8.
[Article] [PubMed]20. Wilde PH, Upadhyay SS, Leong JC. Deterioration of operative correction in dystrophic spinal neurofibromatosis. Spine (Phila Pa 1976) 1994;19:1264–70.
[Article] [PubMed]21. Barker D, Wright E, Nguyen K, et al. Gene for von Recklinghausen neurofibromatosis is in the pericentromeric region of chromosome 17. Science 1987;236:1100–2.
[Article] [PubMed]22. Hsu LC, Lee PC, Leong JC. Dystrophic spinal deformities in neurofibromatosis: treatment by anterior and posterior fusion. J Bone Joint Surg Br 1984;66:495–9.
[Article] [PubMed]23. Park BJ, Hyun SJ, Wui SH, Jung JM, Kim KJ, Jahng TA. Surgical outcomes and complications following all posterior approach for spinal deformity associated with neurofibromatosis type-1. J Korean Neurosurg Soc 2020;63:738–46.
[Article] [PubMed] [PMC]24. Song Q, Leng J, Qu Z, et al. Treatment of scoliosis with one-stage posterior pedicle screw system by paraspinal intermuscular approach: a minimum of two years of follow-up. Orthop Surg 2022;14:3100–10.
[Article] [PubMed] [PMC]